Previous research (2016-2023)
Previous research focused on:
- which of the many natural enemies that attack African boxthorn in the native range are the most appropriate candidate biological control agents for this weed,
- undertaking comprehensive testing to demonstrate that the biocontrol agent(s) do not pose a threat to non-target plants and
- if approved by the authorities, piloting/trialling releases of the agents across the range of the weed in Australia.
This research was undertaken as part of two projects led by AgriFutures Australia and primarily supported by funding from the Australian Government Department of Agriculture, Water and the Environment as part of its Rural R&D for Profit programme rounds 2 and 4:
- Round 2: Biocontrol solutions for sustainable management of weed impacts to agricultural profitability (2016-2020)
- Round 4: Underpinning agricultural productivity and biosecurity by weed biological control (2019-2023)
More information about the AgriFutures projects can be found here: https://agrifutures.com.au/wp-content/uploads/2022/04/AgriFutures_Weeds-newsletter_Autumn-2022.pdf
African boxthorn was endorsed as a target for biological control in August 2016 by the national Invasive Plants and Animals Committee (such endorsement is now the responsibility of the Environment and Invasives Committee and is required in Australia before permission to release a biological control agent can be granted).
The overlapping Round 2 and 4 projects were designed to complement each other and included the following main activities:
- Define management goals:
- what stakeholders would like biological control to achieve.
- Undertake literature searches:
- to gather information on the target weed, its distribution and its known natural enemies.
- Identify appropriate region(s) to source candidate biological control agents:
- Bioclimatic modelling; the climate of the native and invaded ranges of the target weed are characterised and compared using a distribution modelling tool. By comparing meteorological data from the different regions, specific area(s) of the native range can be identified where potentially best climatically suited candidate agents will be found.
- Genetic study; molecular characterisation of the target weed is used to determine the weed genetic structure which helps identify the area(s) of the native range where the weed originates from.
- Conduct native range surveys to identify candidate agents:
- Some level of quantification of the damage caused by the natural enemies of interest is performed while surveying in order to assist selection of the most promising agents for further investigations.
- Test the host-specificity of promising candidate agents:
- Host-specificity testing is necessary to determine the potential range of plants (hosts) which will be attacked by the candidate agent in Australia. Testing methods are always tailored to the particular agent and seek to understand the potential range of plants that would be accepted by the agent after its release in the field. Testing follows a centrifugal phylogenetic approach that concentrates on closely related plant species to the target weed.
- Prepare and submit applications for release of suitable candidate agent(s) to the relevant authorities, provided testing results demonstrate that non-target plants in Australia would not be at risk.
- Establish monitoring plots and gather baseline vegetation data prior to the release of agents.
- Once permission to release an agent was obtained, trial releases of the agent into the field across key areas to determine optimum methods for establishing the fungus.
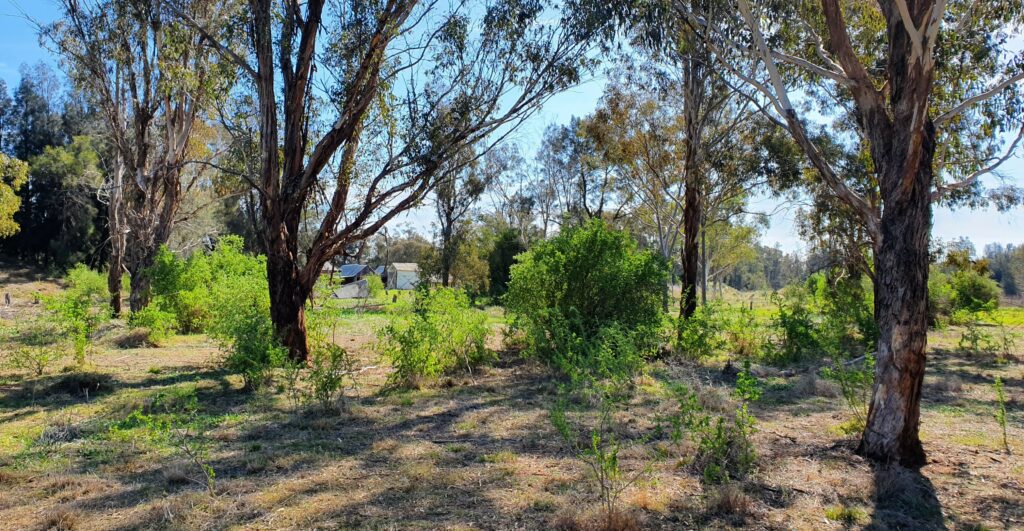
Widespread infestation of young African Boxthorn under native tree canopies, Forbes NSW.
Achievements of Rural R&D for Profit round 2 project
Biocontrol solutions for sustainable management of weed impacts to agricultural profitability (2016-2020)
Undertake a literature review on taxonomy and distribution of African boxthorn and known natural enemies of the weed in the introduced and native ranges
Taxonomy
Species within the genus Lycium are highly plastic, so delimiting and identifying species within the genus with morphological characters can be difficult (Levin et al. 2006; Venter 2000). This is especially relevant to regions like southern Africa that has a high diversity of species in this genus. Morphological variation within Lycium ferocissimum is substantial (Venter, 2000), perhaps influenced by the broad range of climatic and environmental conditions across its distribution in South Africa. Identification of this species in the field is therefore challenging.
Morphometric analyses across L. ferocissimum and other Lycium species in South Africa were undertaken during this project and this research is now published in McCulloch et al. (2020). No leaf or floral characteristics unique to L. ferocissimum were identified, making morphological identification of the species problematic. This is not an issue in Australia, because there are only four other Lycium species present, outside of cultivation, all with restricted distributions: the native L. australe and the naturalised L. barbarum, L. chinense, and L. afrum of Eurasian origin. To ensure the correct Lycium species was surveyed for candidate biocontrol agents, L. ferocissimum individuals for which the identity was confirmed with genetic analyses, were permanently tagged.
Distribution
In Australia, L. ferocissimum is widespread in coastal to semi-arid inland habitats and islands of southern Australia, with records from every jurisdiction (GBIF.org, 24th July 2018; Fig. 1). It is found predominantly in the southern part of the Australian continent in coastal and island situations (except Queensland). Inland, L. ferocissimum is abundant in areas of New South Wales, Victoria and South Australia, where it is a common weed of semi-arid pastures and rangelands and is often found growing along dry stream beds. It has a lesser, but significant presence in south-east Queensland, southern Western Australia, and Tasmania.
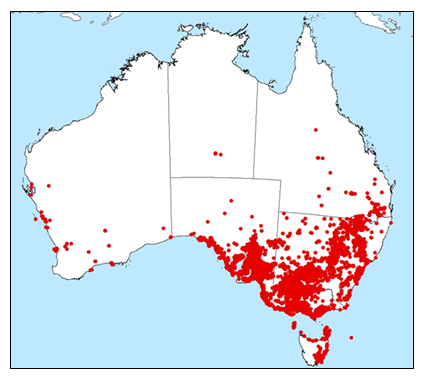
Figure 1. Current distribution Lycium ferocissimum (African boxthorn) in Australia (GBIF.org 24th July 2018).
Lycium ferocissimum has a relatively widespread distribution in South Africa (Venter 2000); however, most herbarium records are from the Eastern and Western Cape provinces. Its distribution overlaps with several morphologically similar and closely related species with which L. ferocissimum hybridizes.
Natural enemies
A comprehensive review of the literature was undertaken in late 2016 to identify potential agents already recorded on L. ferocissimum in the native range, South Africa, and determine if any of these are already present in Australia. At the time of the review, 4 fungi and 13 insects had been recorded on L. ferocissimum in Australia. None of these have been recorded in South Africa. We found records of only one pathogen, the rust fungus Puccinia rapipes, and eight insect species on L. ferocissimum in South Africa. Among these, the rust fungus, tortoise beetles, Cassida distinguenda, Cassida lycii and Cassida sp., and the mirid bug, Schuhistes lekkersingia appeared to be specific enough to warrant further study as potential biocontrol agents.
Defined goals for management of African boxthorn
In order to better understand the impacts of L. ferocissimum on Australian natural ecosystems and agriculture, and to guide the selection of candidate biocontrol agents, a national online stakeholder survey was conducted. Agricultural, community and environmental stakeholders were invited to complete a survey entitled ‘African boxthorn management objectives’, using the online survey platform SurveyGizmo between May and July 2017. Results from this survey are published in Ireland et al. (2019b).
Of the 239 responses received, respondents primarily identified as natural resource managers, specialists or extension officers (61%). Community, conservation or other interest group members (22%), agricultural landholder or graziers (19%), and agricultural land managers, agronomists or extension officers (7%) made up the remaining respondents, with some respondents identifying with more than one group. A range of reasons were provided by respondents as to why L. ferocissimum was problematic, aside from it being difficult (79% agricultural and 86% environmental) and costly (78% agricultural and 84% environmental) to control in both the agricultural and environmental sectors (Ireland et al. 2019b).
Many respondents (79%) agreed that biocontrol solutions for L. ferocissimum would be useful, particularly in difficult to access and environmentally sensitive areas, and when adopted as part of an integrated weed management framework. Respondents’ top six management objectives to which biocontrol needs to make a significant contribution were a reduction in:
- incidence of new infestations,
- management costs,
- negative impacts on native biodiversity,
- weed seed bank over time,
- the need for follow up manual control,
- herbicide use.
Nominating African boxthorn as a biocontrol target
The Invasive Plants and Animals Committee (IPAC; now the Environment and Invasives Committee; EIC), a cross-jurisdictional sectoral sub-committee of the National Biosecurity Committee, endorsed L. ferocissimum as a biocontrol target in August 2016. Unbeknownst to us, the nomination had been prepared and submitted to IPAC by DPIPWE, Tasmania prior to the commencement of the project. One of the members of the project team, however, approached the authors of the nomination document to assist in updating and reformatting the information into a review paper on L. ferocissimum for publication (Noble et al. 2021).
Conducting genetic analysis on samples of African boxthorn from different regions in Australia and the native range
Putative L. ferocissimum (i.e. tentatively identified morphologically in the field) samples were collected across its native range in South Africa and introduced range in Australia. A first set of genetic analyses (three chloroplast markers, one nuclear marker) were conducted in order to: (a) confirm plant identity, (b) assess genetic structuring across the native and invaded ranges (to explore the provenance of the invasive lineage), and (c) assess evidence for hybridisation between L. ferocissimum and other Lycium species that occur in Australia. Results from this study are published in McCulloch et al. (2020).
All samples collected in Australia were confirmed as L. ferocissimum, with no evidence of hybridisation with any other Lycium species. Ten samples from South Africa putatively identified in the field as L. ferocissimum were genetically characterised as different (unidentified) Lycium species. Nuclear and chloroplast genetic diversity within L. ferocissimum across both South Africa and Australia was low, with no evidence of genetic structure. The lack of any detected genetic diversity and structure across L. ferocissimum in South Africa made inferring the introduction history of the invasive lineage challenging. However, one of the two chloroplast haplotypes found in Australia was identified in South African material only from plants collected in the Western Cape Province, near Cape Town. The other common chloroplast haplotype identified in Australia was also found in plants from this area, as well as other regions in South Africa. This suggested that the region around Cape Town may be the provenance of the invasive Australian lineage, though the possibility that L. ferocissimum was introduced to Australia from multiple localities could not be excluded.
A second set of genetic analyses using the more powerful next generation sequencing approach, genotyping-by-sequencing (GBS), was performed to better characterise the likely origins of Australian genotypes of L. ferocissimum (Gurdasani et al. in preparation). We compared 3409 SNPs across 442 L. ferocissimum samples from 64 localities across the entire distribution of the species in South Africa and Australia. Clear geographic genetic structuring was detected across South Africa, with distinct populations across the Eastern and Western Cape provinces. Our analyses indicated that the invasive L. ferocissimum plants in Australia originated from the Western Cape province, with plants from near Malmesbury (in the northern part of the species’ distribution) the closest genetic match to the Australian samples. Samples from Australia had similar levels of genetic diversity as those from South Africa, but there was no evidence of genetic structure across Australia. Our results suggested that the search for candidate biocontrol agents for L. ferocissimum should be focused in the Western Cape province.
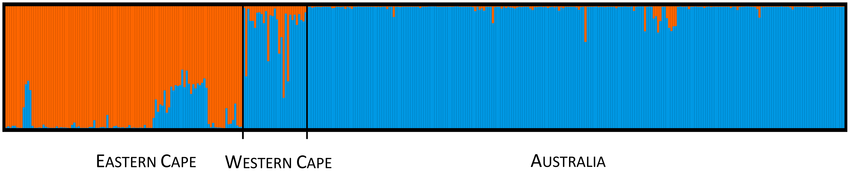
Australian Lycium ferocissimum plants are genomically most similar to plants from the Western Cape. STRUCTURE plot for L. ferocissimum samples collected from across its native distribution (South Africa) and introduced range (Australia). Each vertical bar represents an individual, with colour representing the inferred genomic cluster (McCulloch et al. 2023).
Undertertook bioclimatic models to identify optimal locations and conduct native range surveys and host-specificity tests for potential biocontrol agent(s) and import at least one potential agent in quarantine
Bioclimatic modelling
Bioclimatic models using the CLIMEX were developed (Kriticos et al. 2021). The simpler Match climates model was used to identify where to focus the search for candidate biocontrol agents in South Africa. It showed that L. ferocissimum is climatically well-matched to the Mediterranean and dry arid and semi-arid climate zones in South Africa, which accords with the rangelands in which it is an invasive problem in Australia.
Surprisingly there were little data on the climatic requirements and physiology of L. ferocissimum in the literature to inform the more sophisticated Compare Locations model. An experiment was thus conducted to measure the growth rate of L. ferocissimum at different temperatures to refine temperature growth-related parameters for the model. From this model, we extracted the Monthly Growth Index values in South Africa for guiding when and where to survey for natural enemies on L. ferocissimum. Similar values were extracted for Australia to guide when and where biocontrol agents should be released, so that L. ferocissimum is actively growing at the selected sites at the time of release, in order to increase chances of establishment.
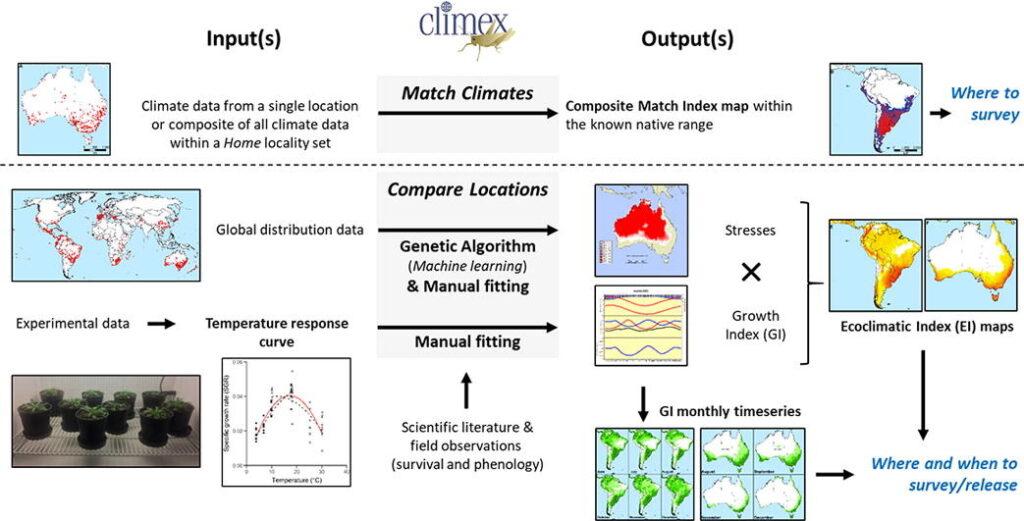
Framework for integrating CLIMEX ecoclimatic modelling into the exploration (native range surveys) and deployment (release strategies) stages of classical biological control programmes. The dotted line separates the simple Match Climates modelling approach from the more complicated but robust Compare Locations modelling approach (Kriticos et al. 2021).
Native range surveys
Pathogens
A comprehensive survey for diseases on L. ferocissimum was performed in October 2017 at 28 sites across the Eastern (13 sites) and Western (15 sites) Cape provinces of South Africa. Results from this survey are published in Ireland et al. (2019a). Disease symptoms caused by the rust fungus Puccinia rapipes were observed on L. ferocissimum at 4 of the 13 sites in the Eastern Cape and 10 of the 15 sites in the Western Cape. The rust fungus was not observed on any other Lycium species. The most severe rust symptoms were observed on L. ferocissimum at coastal sites in the Western Cape. Disease symptoms of any other primary pathogens were not observed at any of the sites surveyed.
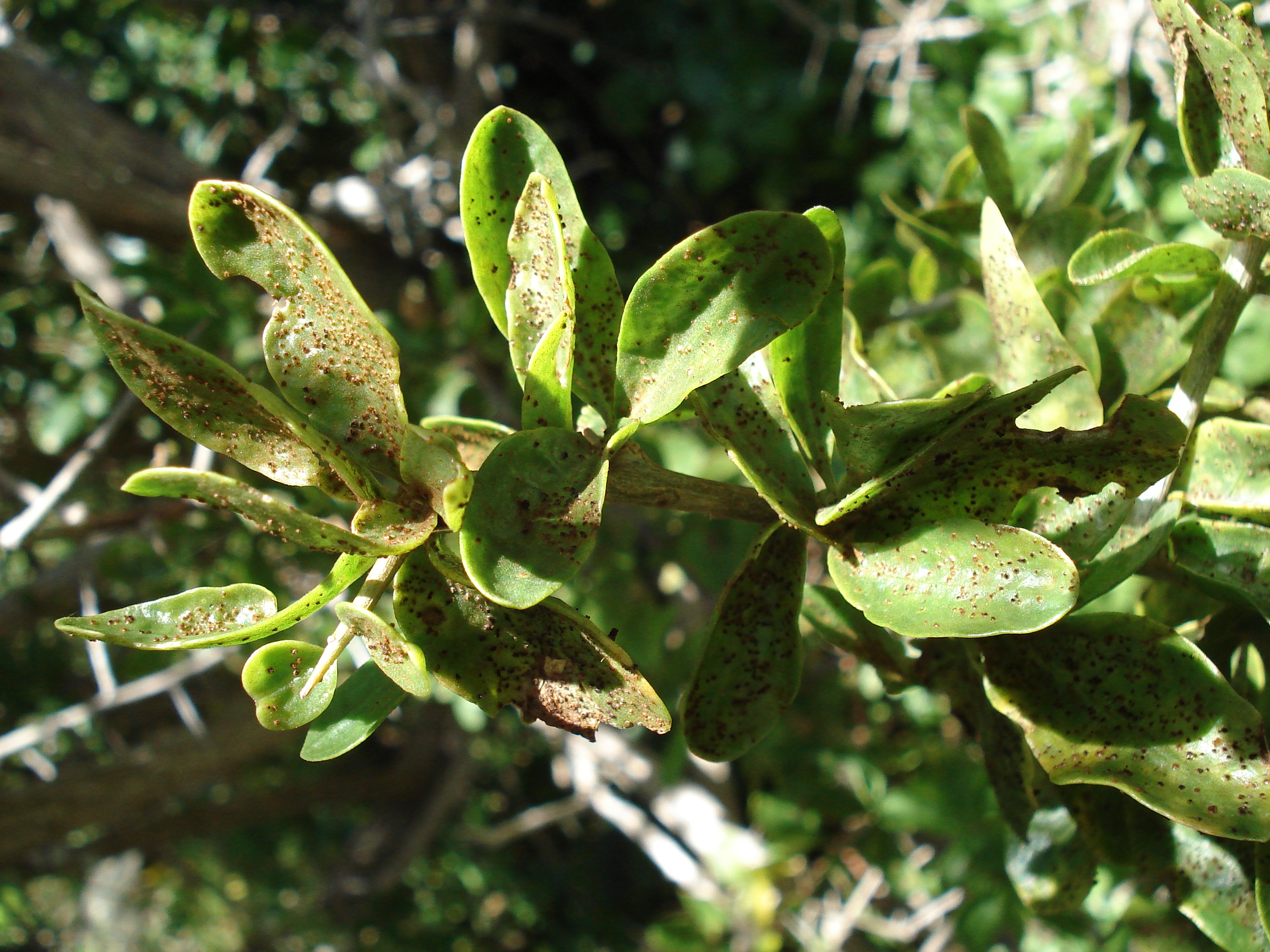
The rust fungus Puccinia rapipes on African boxthorn in South Africa (photo courtesy of Alan Wood PPRI-ARC).
Two sites in each of the Western and Eastern Cape provinces were also visited multiple times between November 2016 and October 2017 to source material of P. rapipes to establish cultures in the Australian containment facility and track life cycle development. On each field visit, rust life cycle stages and evidence of any other disease symptoms were recorded. Uredinia, telia, spermogonia and aecia were observed on L. ferocissimum at both sites where the Eastern and Western Cape during the course of these visits, confirming that the rust fungus is a macrocyclic and autoicous (no alternate host) rust fungus (Ireland et al. 2019a).
Insects
Native range surveys for potential insect biocontrol agents on L. ferocissimum were performed over a two-year period in the Eastern Cape and Western Cape provinces of South Africa. In total, 96 insect species comprising 1315 individuals were collected from both provinces (Chari et al. 2020). Of the 96 species, three species, the leaf-chewing beetles Cassida distinguenda Spaeth (Chrysomelidae) and Cleta eckloni Mulsant (Coccinellidae) and the leaf-mining weevil Neoplatygaster serietuberculata Gyllenhal (Curculionidae) were prioritised as potential biocontrol agents based on their distribution, abundance, preliminary biology studies, pilot in-field host-specificity studies (in South Africa) and feeding preference.
Importation of candidate agents into quarantine
Pathogens
An accession of P. rapipes from the Western Cape Province of South Africa was imported in quarantine in Australia early 2017, but a culture could not be established because teliospores were dormant. Later in 2017 two additional shipments of rust-infected material were imported; an accession from the Eastern Cape Province in August and another accession from the Western Cape Province in October. Material from both accessions contained urediniospores that readily germinated, and a culture of each accession was established in quarantine. These two accessions were purified to generate single-uredinum isolates, bulked-up and used in a series of experiments.
Insects
Accessions of C. eckloni from the Eastern Cape and Western Cape provinces were imported into Australian quarantine in May 2019 and February 2020, respectively.
Host-specificity tests for potential biocontrol agents
The proposed list of non-target species for host-specificity testing of candidate biocontrol agents for L. ferocissimum was submitted to DAWE in December 2018 for posting on their website for feedback (Ireland et al. 2018).
Pathogens
A preliminary host-specificity study using two purified isolates of P. rapipes, from the Eastern and Western Cape provinces of South Africa, was performed in quarantine in Australia (results published in Ireland et al. 2019a). The experiments comprised two different chloroplast haplotypes of L. ferocissimum identified in Australia (McCulloch et al. 2020) and seven species closely related to the weed that occur in Australia. The L. ferocissimum haplotypes and the three Lycium species of Eurasian origin tested ‒ L. barbarum, L. chinense and L. ruthenicum ‒ were found to be susceptible to both isolates of P. rapipes used, while the Australian native L. australe was resistant. The three more distantly related species to L. ferocissimum tested were immune to the fungus: Hyoscyamus albus, Hyoscyamus aureus and Solanum aviculare. The susceptibility of Goji berry (L. barbarum) to P. rapipes was further confirmed by our collaborators in South Africa in a study performed under natural conditions.
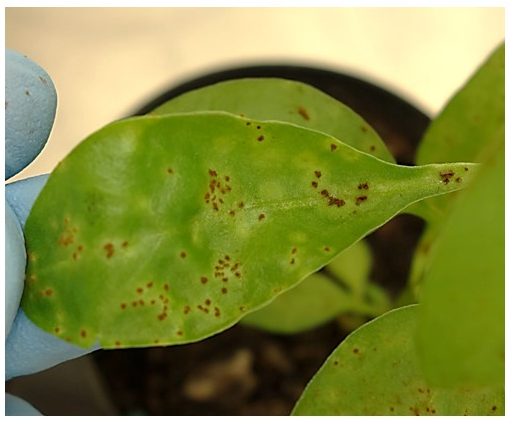
A leaf of African boxthorn infected by the rust fungus Puccinia rapipes.
To better appreciate the implications of such results, further testing was suspended, and we undertook extensive stakeholder consultation. We first reviewed the known and potential economic and social importance of non-native Lycium species propagated and sold within Australia, ascertained by review of the literature, online searches and discussions with stakeholders. We then further consulted growers and retailers of Goji berry to identify possible concerns they would have if a weed biocontrol agent was to be released that could infect their Goji berry plants. With this background information provided, we asked the national Plant Health Committee (PHC) for advice on whether it would be acceptable for an exotic weed biocontrol agent targeting African boxthorn in Australia to also infect Goji berry. This committee is responsible for reviewing applications for release of weed biocontrol agents that are submitted via DAWE and thus is in the best position to provide initial advice on this issue. This consultation process indicated that Goji berry is an extremely low value and volume plant in Australia, and overall growers, wholesalers and retailers were not particularly concerned about the possible release of a biocontrol agent for the L. ferocissimum that would also affect Goji berry. Based on the responses received, including those from PHC, it was decided to resume work with P. rapipes.
Comprehensive host-specificity testing in quarantine began in September 2019 using one of the purified isolates (ex. Western Cape). The test list comprises a total of 31 closely related, non-target species in the family Solanaceae that occur in Australia (ornamental, weed and native). Most species are tested in at least two separate experiments using different accessions of each plant species, with L. ferocissimum plants used as positive controls in all experiments. Testing is on-going and thus far, 11 experiments have been conducted comprising 17 different species (Ireland et al. in preparation). Similarly, to results of the preliminary study, all the Lycium species non-native to Australia included in these experiments to date, including the target weed L. ferocissimum and the Eurasian Goji berries L. barbarum and L. ruthenicum were found to be susceptible to P. rapipes. In contrast, all accessions of the Australian native Lycium australe tested were found to be immune to the fungus, while all other species tested were either immune or displayed various levels of resistance to P. rapipes.
The host-specificity testing of the African boxthorn rust fungus, Puccinia rapipes, and the release application were completed during the reporting period. The release application was submitted to the Department of Agriculture, Water and the Environment on 27 November 2020.
Insects
Pilot in-field host specificity tests with the three promising candidate agents (C. eckloni, N. serietuberculata, C. distinguenda) were conducted on selected test plants at three sites in the Eastern Cape Province in South Africa: L. oxycarpum, L. barbarum, Solanum melongena (eggplant). There was generally very minimal or no spill over of the insects on the non-target plants. At only one of the sites, three C. eckloni and one C. distinguenda individuals were observed resting on L. oxycarpum and S. melongena, but without any signs of feeding.Preliminary host-specificity testing with C. eckloni and C. distinguenda was also conducted in a glasshouse at Rhodes University in South Africa. These tests showed that the insects have a host range restricted to the genus Lycium. Adult feeding and oviposition were recorded on L. barbarum, and both insects were able to complete their life cycle on this species.
Among the three prioritized agents, C. eckloni from a population in the Eastern Cape province was first imported into an Australian quarantine facility in February 2019 (and again in April and Mary 2019, to bolster colonies) (Fig. 8). Comprehensive no-choice host-specificity tests with this leaf-feeding beetle revealed that it feeds and reproduces on the three Lycium ‘goji berry’ species (L. chinense, L. barbarum and L. ruthenicum) as well as the native L. australe to the same extent observed on L. ferocissimum (Fig. 9).
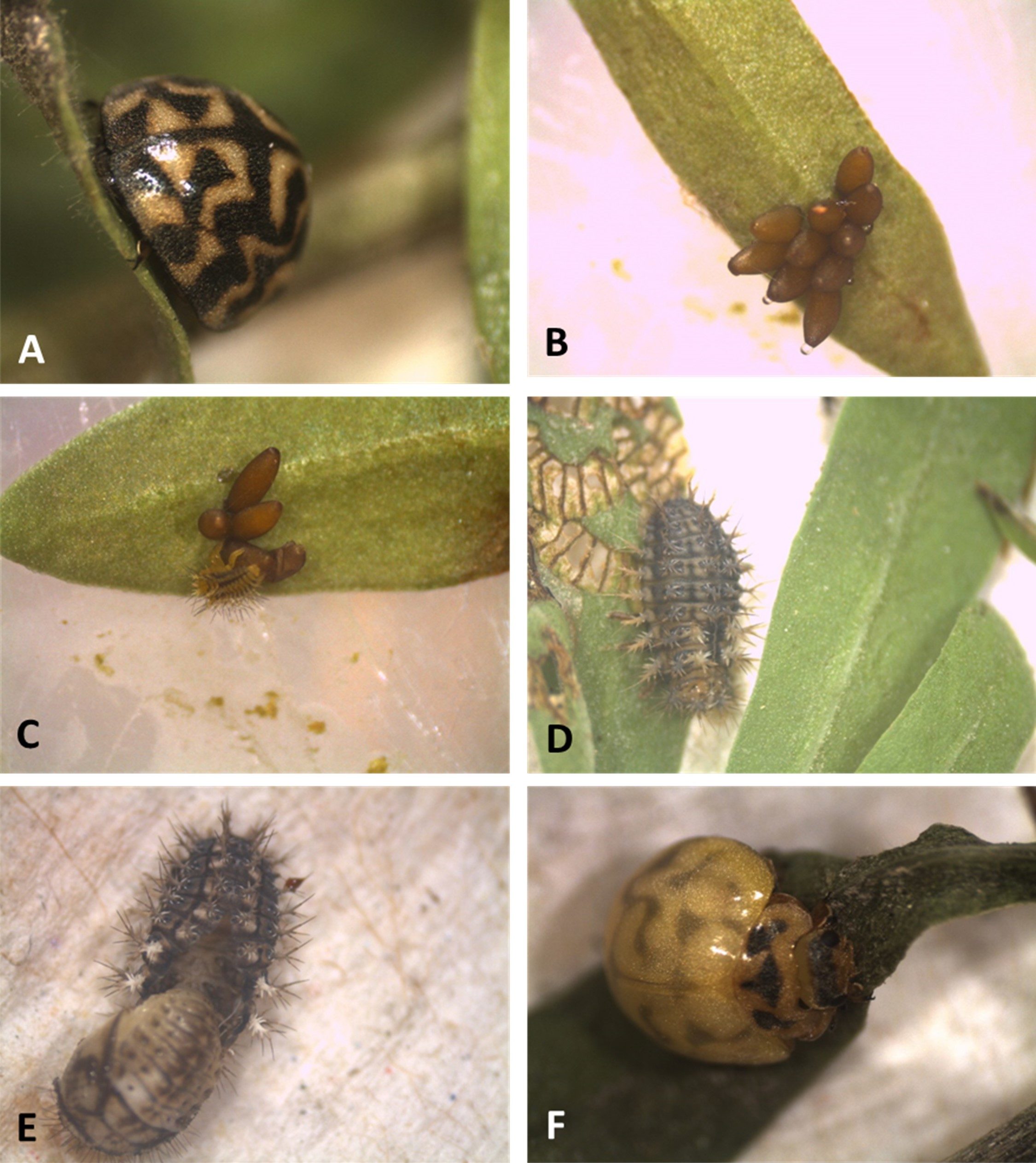
Cleta eckloni. (A) adult, (B) eggs which are laid in clusters on the abaxial side of the leaves, (C) 1st instar larvae on top of eggs, (D) larvae with diagnostic branched processes on top of the damaged leaf with the distinct feeding pattern on the leaf, and (E-F) larvae of the last instar stage and newly pupated adult from the last instar stage (photo courtesy of Evans Mauda, Rhodes University).
As part of the investigations on C. eckloni we noticed morphological and molecular variation in this taxon in key diagnostic features between populations from the Eastern Cape and Western Cape provinces. This suggested that this taxon was perhaps a complex of cryptic species. In order to explicitly test this, we have commenced molecular characterisation of this species and, in Feb 2020, we imported a population of this species from the Western Cape to repeat the host-specificity testing undertaken with the Eastern Cape population. This testing will be part of the new Rural R&D for Profit project (Agrifutures Australia Project number: PRJ-12377). If the test results are similar to those with the Eastern Cape population, further testing with this insect will be discontinued and we will shift our focus to other candidate insect agents identified during native range surveys.
Explored options for integration of biocontrol with other management techniques
Long term effective control of L. ferocissimum requires a combination of treatments over many years due to the capacity of the species to regenerate from rootstock, stems and seed. Lycium ferocissimum seed is dispersed predominantly by birds and other fauna, and potential for re-infestation of sites from outside sources should be considered in management planning.
Physical control techniques
Physical control of L. ferocissimum includes winching, pulling, bulldozing, stick raking, blade ploughing and cultivation (Noble and Rose, 2013). These techniques are best used when L. ferocissimum plants are not carrying seed (or are carrying minimal seed). Otherwise, fresh seed is likely to be deposited into freshly disturbed soil. Winching and pulling are the lowest impact physical control techniques for situations where disturbance is a concern, such as where L. ferocissimum is growing within native vegetation. Bulldozing, stick raking and blade ploughing are suitable in less sensitive landscapes (e.g. pasture), and provide a rapid control method for moderate to heavy infestations.
Successful management of L. ferocissimum using the above techniques is dependent on follow-up application of herbicide. This includes cut-stump technique with immediate application of herbicide for any remaining base/roots after winching and pulling. For all physical control techniques, there is a need to return periodically and carry out foliar spray application and/or machine-based cut stump treatments until there is no regrowth or seedling presence (Noble and Rose, 2013).
Chemical control techniques
Chemical control of L. ferocissimum uses techniques including foliar spraying, cut-stump application (including mechanical cut-stump), stem injection, stem scrap or frilling (e.g. using chisel cuts), basal bark application, and soil-root zone application. Glyphosate, triclopyr, picloram, aminopyralid, hexazinone and tebuthiuron have all been trialed in the chemical control of L. ferocissimum. Appropriate formulations, mixes and applications of these chemicals are details in Noble and Rose (2013) and Noble et al. (2021).
Biocontrol
Biocontrol is likely to be an important component of landscape scale management of this species. The rust pathogen is likely to establish and perform optimally in wetter or more humid parts of the L. ferocissimum distribution in Australia, and in wetter years. Sites that fit these criteria might make good nursery sites, where the agent could initially establish and from where natural or assisted dispersal of the agent could occur.
Integration of management tactics
At the landscape scale, minimising the disruptions of biocontrol agents by other control tactics (e.g. avoiding spraying plants in nursery sites with herbicides) will require coordination among land managers recommending/deploying management tactics for L. ferocissimum. In addition, there are likely to be many circumstances where the pathogen (and other biocontrol agents) may act in concert with physical and chemical management. For example, it could be of value to trial the use of the pathogen as a follow-up treatment to control recruiting seedlings in place of herbicide applications. Similarly, the timing of releases of the pathogen with periods of regrowth of the weed following physical treatment, may also aid the integration of biocontrol to be another chronic stressor for this weed.
Achievements of Rural R&D for Profit round 4 project: Underpinning agricultural productivity and biosecurity by weed biological control (2019-2023)
Pre-release monitoring of vegetation at selected future release sites of biocontrol agent(s)
The overall aim of vegetation monitoring at prospective biocontrol agent release sites was to characterise the population structure (e.g., plant density and size distribution) and abundance (e.g., biomass, foliage cover) of the focal weed and associated vegetation prior to release of a biocontrol agent. Pre-release monitoring establishes a baseline of vegetation condition against which the impacts of the biocontrol agent on the host weed can be assessed over time.
Between July and November 2020, 14 permanent monitoring plots (20 m × 20 m; 400 m2) were established across 7 sites in 3 regions in south-east Queensland, southern NSW and the ACT. We sampled a range of invaded habitats, including highly degraded and intermittently grazed remnant grassy Eucalyptus woodlands with an understorey of predominantly non-native pasture grasses, pine plantations, grazed pastures and roadsides. This will allow us to ascertain which, if any, habitat features are most strongly associated with African boxthorn population growth and, in the future, performance of the biocontrol agent(s) released. Within each plot, pre-release baseline data were gathered on African boxthorn population demographics using methods developed in April 2020 in the ACT.
Altogether (i.e. summed across the 14 plots), we identified, tagged and measured size and reproduction parameters for 258 plants. This data synthesis exercise revealed significant variation in estimated African boxthorn population productivity across the 14 plots, with a mean ± SD of 6.2 ± 5.4 (ranging from 1.2 to 18.7) tonnes per hectare. This variability will enable us to determine whether establishment, spread and impact of released agent(s) on African boxthorn are influenced by plant size, population density and other environmental variables.
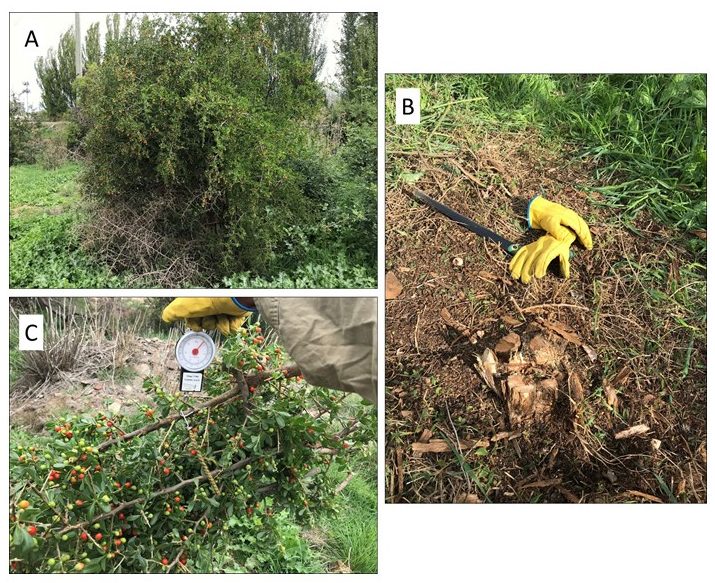
Example of a plant that was destructively harvested by sawing basal stems at ground level, followed by an estimate of above ground wet biomass (kg) weighed using hand-held spring scales. Note the large mass of unripe (green) and ripe (red) fruit but lack of white-pink flowers, thus representing peak fruit output, based on field observations made across the ACT between October 2019 and April 2020.
During March 2021, 11 permanent monitoring plots (20 m × 20 m; 400 m2) were also established at different sites in semi-arid regions of South Australia, consisting of grazed grasslands and grassy woodlands, where African boxthorn invasion causes significant impacts on grazing productivity and the health of native ecosystems. Altogether, since sampling began in July 2020, we established a total of 25 monitoring plots (1 per site) throughout South Australia, south-east Queensland, southern NSW, and ACT, spanning a variety of climate and land use contexts – that is, cool temperate to subtropical to semi-arid grasslands and grassy woodlands used for beef cattle grazing.
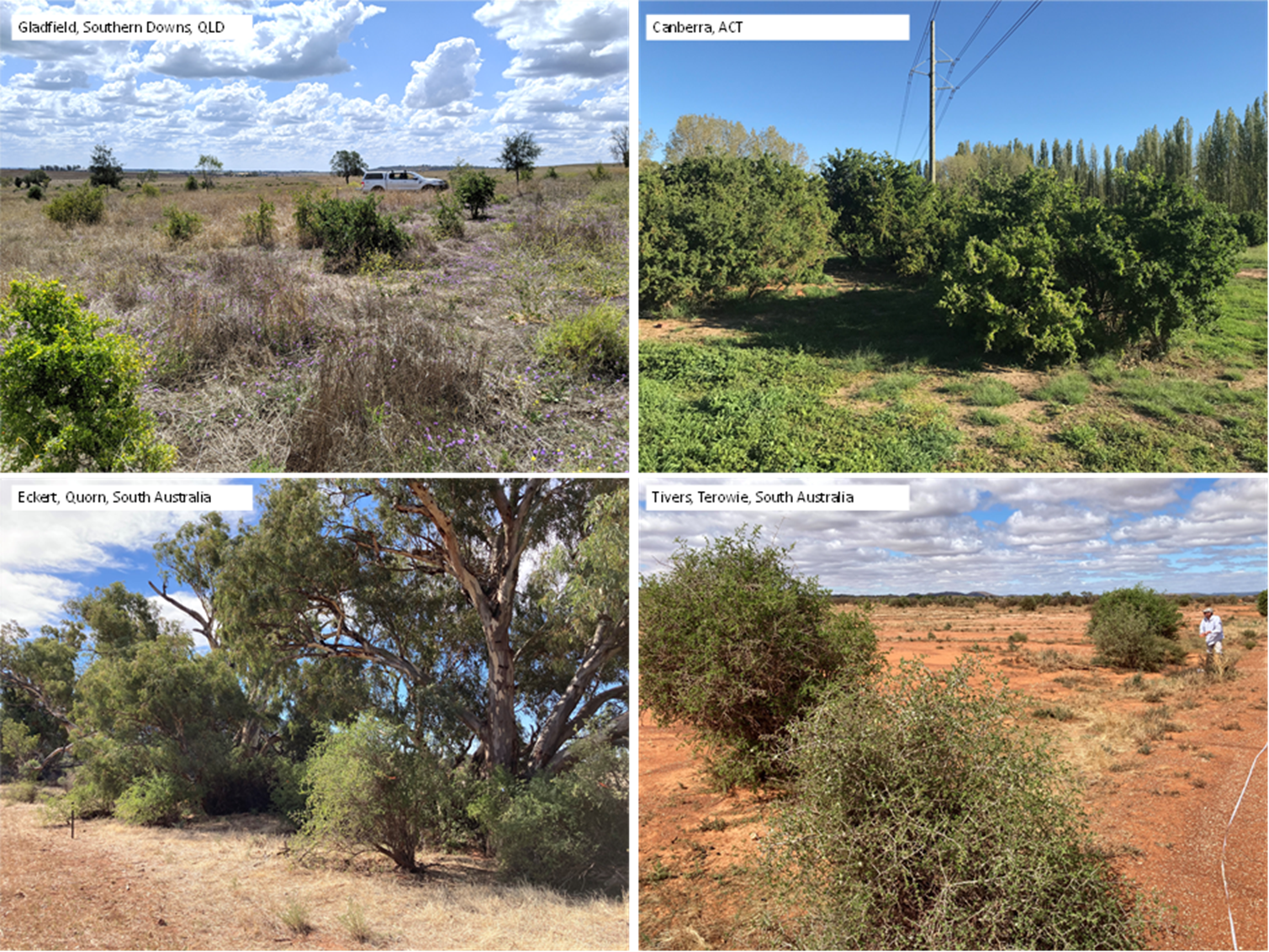
Example of habitat context at four plots sampled in Queensland, ACT and South Australia.
We assessed the abundance of desirable vegetation associated with African boxthorn invasion within 16 of the 25 established plots, and found that the percentage cover of pasture grasses and herbs decreased significantly with increasing cover of African boxthorn, thus confirming previous observations that invasion by this weed can reduce the condition of desirable vegetation. The magnitude of decline was greatest for pasture grasses (5-fold decrease as African boxthorn cover approached 80 %) than herbs (2-fold decrease as African boxthorn cover approached 80 %). These data represent a baseline (i.e., pre-release of biocontrol agent) assessment of vegetation condition against which the benefits of biocontrol agent(s) can be evaluated in the future.
Approved release of the pathogen from biosecurity containment and establishment of a laboratory culture
The Department of Agriculture, Water and the Environment (DAWE) informed CSIRO in May 2021 that a preliminary assessment of the release application for the African boxthorn rust fungus Puccinia rapipes, which was submitted in November 2020, had been completed by a panel within the Department. The panel provided feedback and asked a series of questions. CSIRO answered DAWE questions and made the required minor revisions to the release application in early June 2021. In mid-July 2021, DAWE informed CSIRO that after having considered our responses and revisions they decided to proceed with finalising the preliminary draft risk analysis for P. rapipes and sending it to Plant Health Committee (PHC) for comment. CSIRO was told that all DAWE internal reviewers were comfortable with the draft recommendation to release P. rapipes based on outcomes of the risk analysis. DAWE sent the preliminary draft risk analysis in early August to the national Plant Health Committee for consideration and comments.
The fungal culture is being maintained on African boxthorn seedlings within plant growth facilities at CSIRO’s Black Mountain laboratories. Viable spores are being collected from infected leaves, then dried and frozen for long term storage.
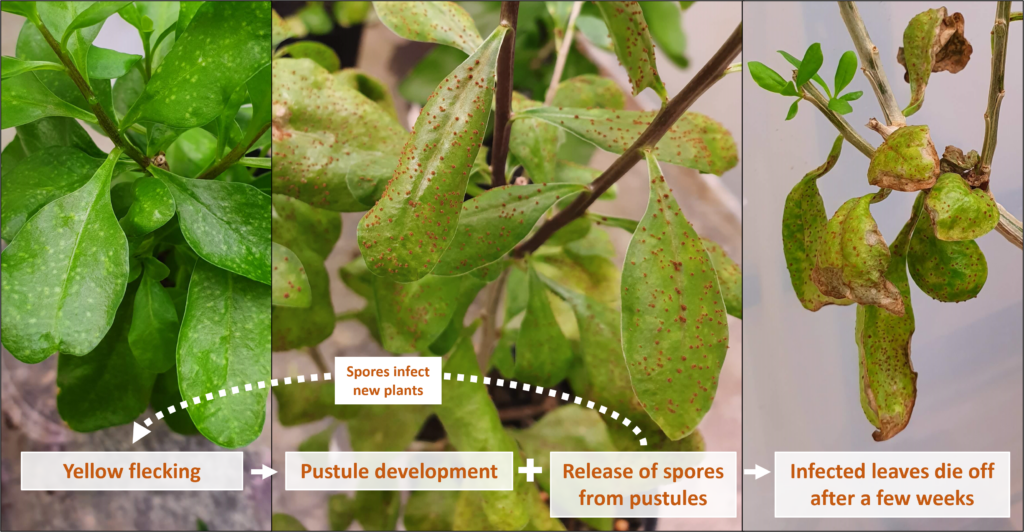
Depiction of disease progression for the fungus on Puccinia rapipes
Experimental optimisation of pathogen release methods in the field
Experimental releases of the fungus were undertaken on dense African boxthorn infestations in the field in the ACT, with the aim of optimising delivery methods to participating stakeholders. The experiments were undertaken between April and June 2022, testing viability of spores which had been removed from freezer storage for various lengths of time, and testing the time of day of release into the field. Upon removal from the freezer, spores were mixed in a 0.1 % Tween solution in a spray bottles, then immediately sprayed liberally across young, healthy foliage of host African boxthorn plants and covered with plastic bags for either 17, 20, or 24 hrs to retain local humidity for spore germination and infection. Post-release monitoring was undertaken after 2 weeks for both trials, with no signs of infection of the host African boxthorn by the fungus. For each of these two field experiments, fungal spores were tested for viability at the time of inoculation – and all batches of spores were found to be viable.
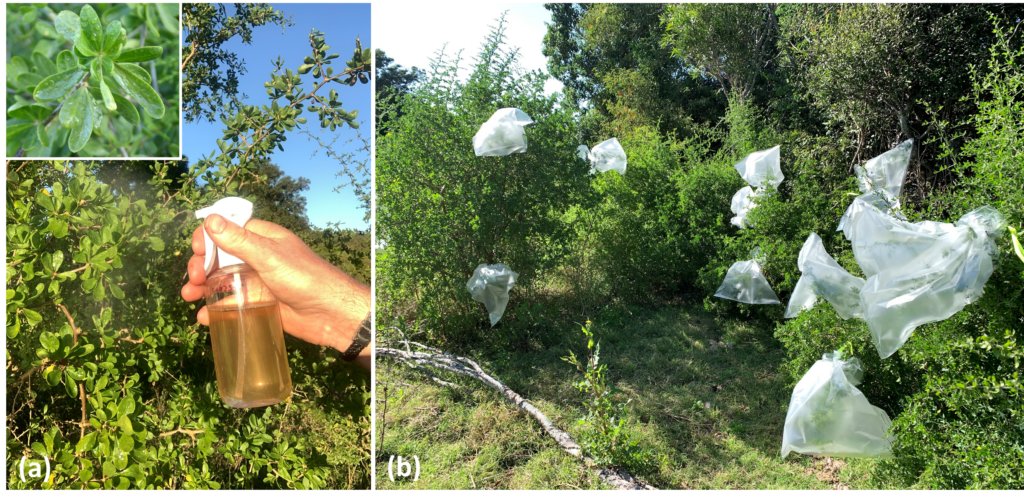
Method used to inoculate naturalised African boxthorn populations with the rust fungus Puccinia rapipes.
It is possible that infection did occur in the field, but cool autumn-winter temperatures in Canberra caused a delay in the onset of visible symptoms. Through a small field experiment set up in August 2022 in the ACT, it was confirmed that the fungus can remain dormant within the leaf tissue (for up to two months) until the ambient temperature become sufficiently high enough to induce disease symptoms.
Pathogen releases at fixed monitoring plots
The fungus was released using the spore suspension and stem-bagging method described above (with stems being bagged for 17 hrs) at two sites in the ACT and one site in QLD. Releases were made in mid-November 2022 once weather had become sufficiently warm (days regularly over 20°C).
At the ACT plots, post-release surveys of fungal infection on African boxthorn leaves were undertaken in February 2023, three months after the fungus was released. Observations were also made of infection on nearby control stems that had not been previously inoculated with the fungus. Altogether, 69 % of the inoculated stems comprised of leaves with visible symptoms of fungal infection, predominantly at the telia stage of reproduction. Some control stems also bared infected leaves, indicating secondary infection whereby the fungus had completed its lifecycle on the first set of inoculated stems that in turn produced spores that spread to nearby stems. As such, it is likely that the fungus has now become established at these sites and is beginning to spread within the local Africa boxthorn population. At the QLD release plot, branches were checked for infection after about 5 weeks, and all branches (100%) had some level of infection present, ranging from a few scattered pustules on a couple of leaves to most leaves covered in infection. After 9 weeks, branches were checked again, and infection was still present on most stems.
Ongoing monitoring will reveal the rate at which the fungus spreads through these local areas.
Community engagement in a pilot mass-release program of the pathogen across Australia
From September 2022 until February 2023, CSIRO researchers launched a pilot biocontrol agent release program in partnership with weed management stakeholders, with the aim of trialling the optimised release protocols for the rust fungus in a variety of landscapes and climate contexts. We selected participants equitably to reflect the broad range of site conditions and across the range of African boxthorn’s core distribution in south-eastern Australia from southern Queensland through to the Eyre Peninsula in South Australia.
Registered participants were sent a biocontrol agent release kit consisting of a vial containing 0.1 ml dried spores of the rust fungus, materials to prepare the agent for release, and a comprehensive set of release and monitoring instructions.
Altogether, 126 biocontrol agent release kits were distributed to 33 participants, drawn from 20 organisations, throughout Queensland, NSW, Victoria, and Tasmania. We also registered participants from Western Australia but permission to import the biocontrol agent to that state had not been granted until December 2022, after which time the daytime temperatures were too high to support fungal infection in the field.
Participants detected the fungus at ~43 % of monitored sites, however many sites had boxthorn plants that were severely stressed due to recent hot/dry weather and had dropped all their leaves – this usually occurred in sites in South Australia. Sites in Queensland appeared to have the most success, with 100 % of QLD sites monitored showing disease symptoms.
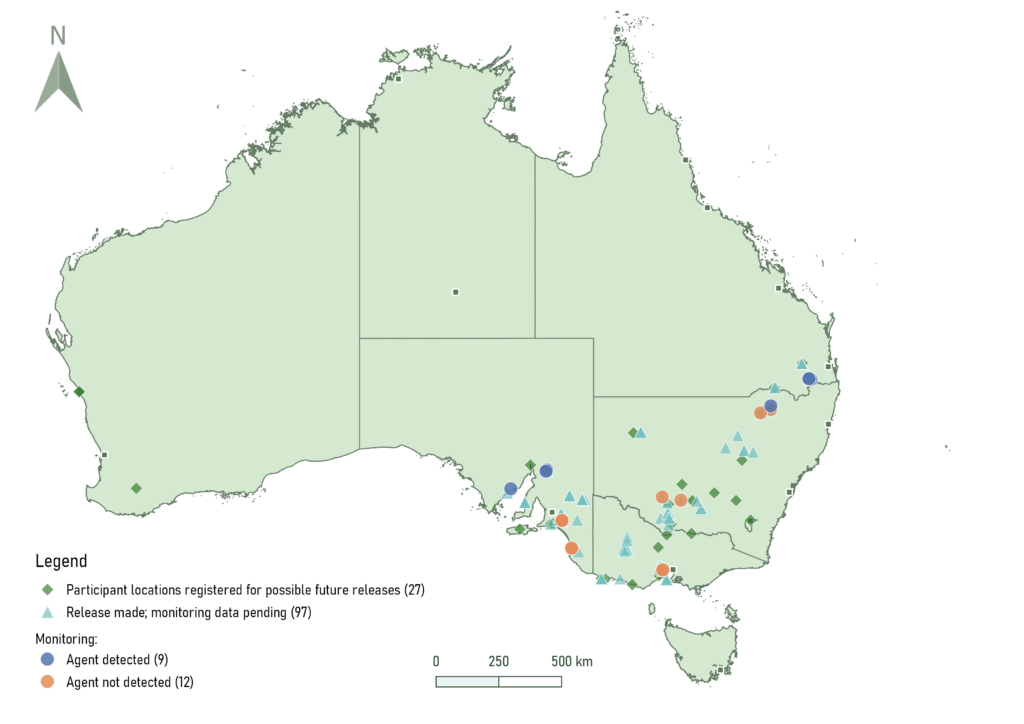
Sites which have been registered for future Puccinia rapipes releases (green diamonds) or have had release made but data on establishment is pending (blue triangles). Monitoring sites where the agent has been released and detected (blue circles) or not detected (orange circles).
Importation of a new candidate agent into quarantine to establish a colony and commence testing
As reported in the final report of the RRnD4P Round 2 project, a new accession of Cleta eckloni was imported to the CSIRO Quarantine facility located in Brisbane from the Western Cape province of South Africa in February 2020. After exporting C. eckloni to Australia, the remaining culture at the Centre for Biocontrol (CBC) at Rhodes University was starting to recover. In March 2020 national lockdowns were imposed in South Africa due to COVID-19. Mid-August 2020 was the first-time CBC staff were allowed to access the mass rearing and research facility. Some plant material had survived the period without maintenance but none of the insect colonies had survived. When domestic travel was permitted, CBC staff commenced field surveys to re-collect samples of C. eckloni, N. serietuberculata and C. distinguenda to restart laboratory colonies. Due to these efforts’, candidate agents were available for import once logistics chains were available.
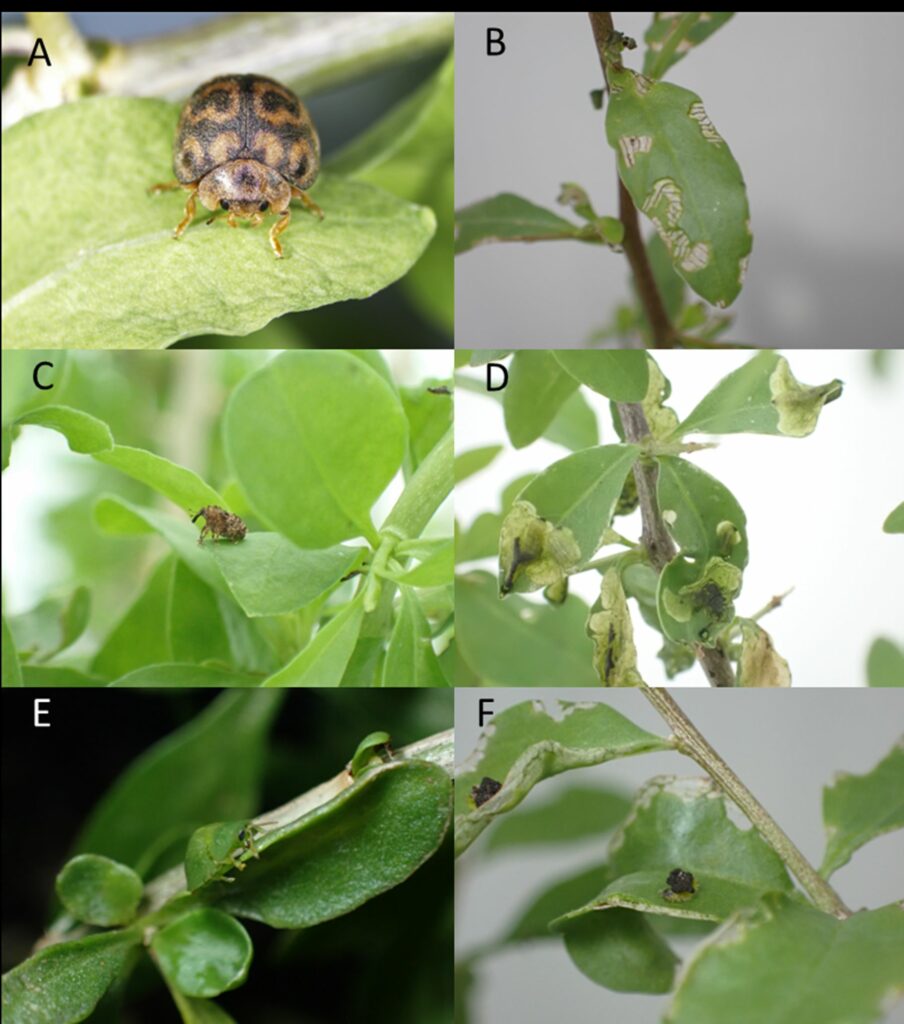
Photos of each of the insects and prioritised for host specificity testing in Australian quarantine. A) Cleta eckloni, B) associated Cleta eckloni feeding damage on African boxthorn, C) Neoplatygaster serietuberculata, D) associated Neoplatygaster serietuberculata larval feeding damage on African boxthorn, E) Cassida distinguenda, F) associated Cassida distinguenda feeding damage on African boxthorn.
A further importation of Cleta eckloni (Western Cape) from South Africa was then attempted in January 2021. Unfortunately, due to delays in logistic transport chains, all insects died in transit. Neoplatygaster serietuberculata was consequently prioritised for further importation attempts as it is more resistant to starvation relative to C. eckloni and thus able to withstand extended freight periods. Several importations of this insect were made in March, May and June 2021. A culture of this insect was maintained in the quarantine facility from March 2021 until November 2022. A third insect Cassida distinguenda was imported into the Brisbane quarantine facility in April and June 2022. A culture of this insect was sustained until January 2023.
Host-specificity testing under quarantine of new imported candidate agents
Neoplatygaster serietuberculata – No-choice testing
The test list comprised of L. australe, which is an Australian native that is distributed across the southern half of Australia, and L. barbarum, L. chinense and L. ruthenicum which are all ornamental and of East Asian origin (Levin et al. 2007). Physalis peruviana and Lycianthes rantonetti, which are ornamentals just outside of the Lycium genus, were also included in a single no-choice test replicate. Both adult and larval feeding were assessed, along with the number of eggs and the number of leaves oviposited on, the number of larvae observed, and the new adults produced were recorded over the duration of the tests.
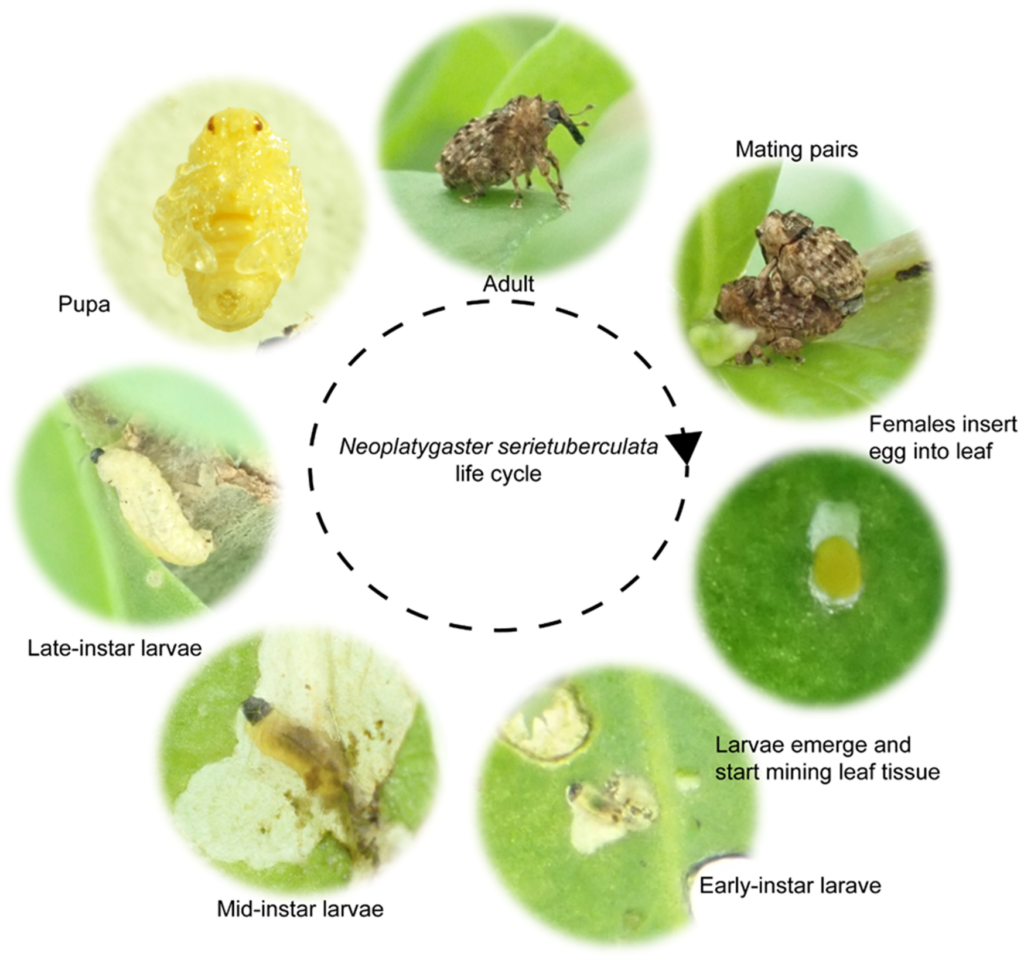
Biology and life cycle of Neoplatygaster serietuberculata, as determined by collaborators at Rhodes University.
Larval feeding in no-choice tests was highest on the native L. australe, followed by L. barbarum and African boxthorn, but there was no significant difference in observed feeding between plant species. Adult feeding was recorded across more of the test plant species, with significantly higher feeding recorded on the native L. australe. The highest number of adults was produced from tests on the focal weed African boxthorn, followed by L. australe and L. barbarum.
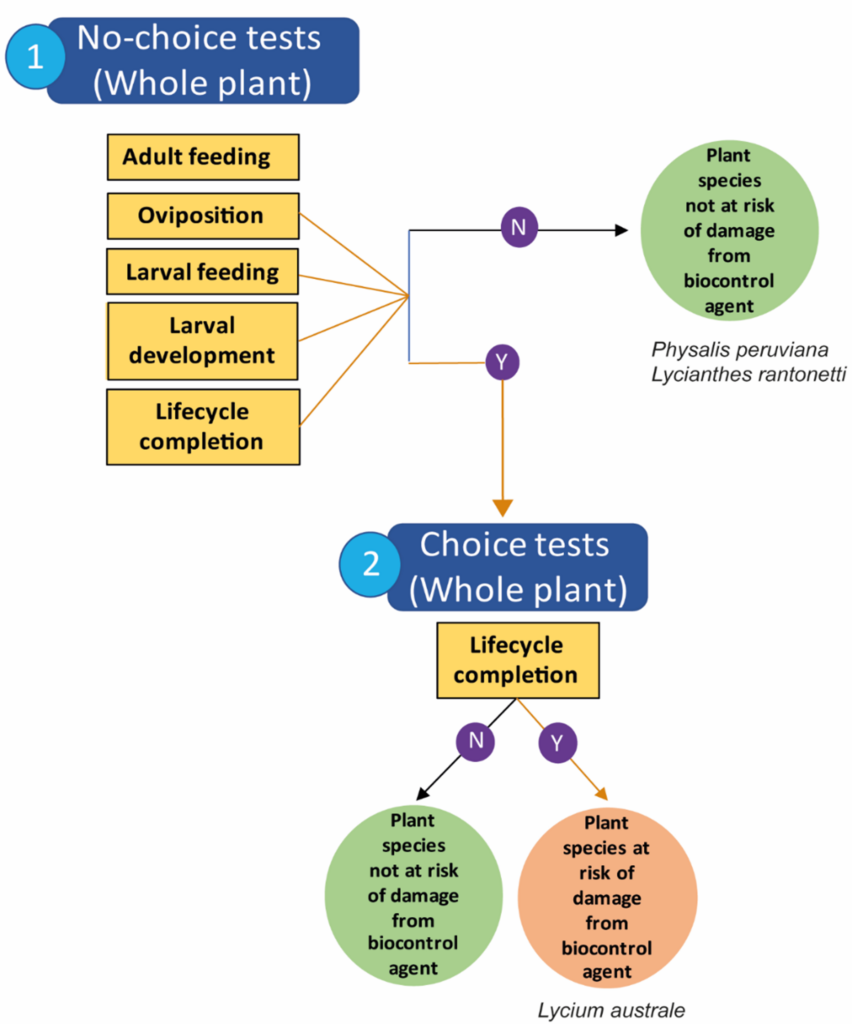
Decision tree for Neoplatygaster serietuberculata biocontrol risk.
Cassida distinguenda – No-choice testing
No-choice tests were performed for L. australe and African boxthorn. Adult insects were placed on each plant of each species after which feeding, survival and oviposition were recorded for two weeks.
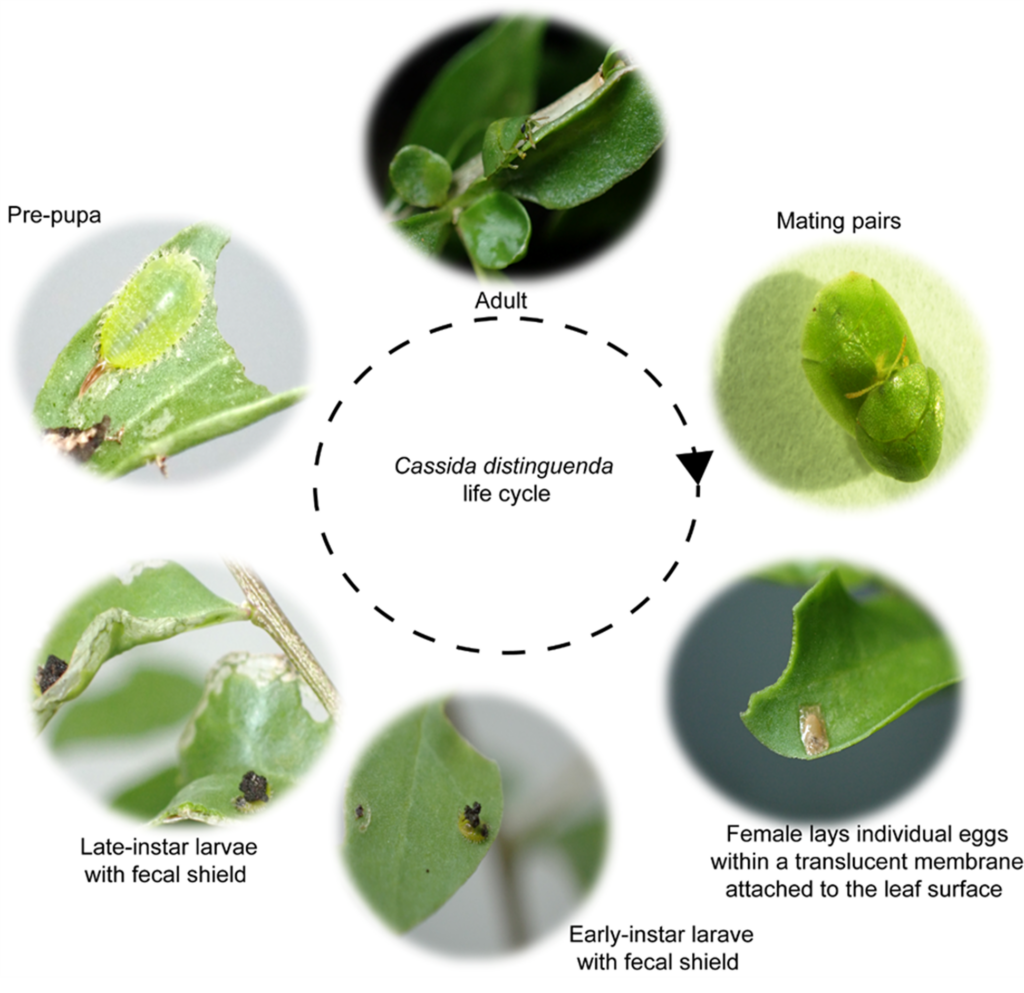
Biology and life cycle of Cassida distinguenda, as determined by collaborators at Rhodes University.
No choice tests revealed that feeding on the Australian native L. australe was higher than that observed on the focal weed African boxthorn for both adults and larvae, but not significantly so. The mean number of adults produced on African boxthorn was significantly higher than L. australe.
Adults produced during no-choice tests were then placed on the same plant species they were produced on for another generation to see if a persistent population could be maintained on L. australe and African boxthorn controls. Feeding, survival and oviposition were recorded for two weeks.
Feeding scores for C. distinguenda adult and larvae remained high across both L. australe and African boxthorn in the continuation trails. More adults were produced on the African boxthorn controls than that observed on L. australe, but the results were not significantly different, indicating that the Australian native is capable of sustaining C. distinguenda in the laboratory context over consecutive generations.
Cassida distinguenda – Choice tests
Paired choice tests of C. distinguenda adults on African boxthorn and L. australe were conducted Inside each cage, the two plants (African boxthorn and L. australe) were placed 10 – 15 cm apart from each other. newly emerged adults were placed on a petri dish in the middle of the cages. The location of the insects within the cage was recoded as well as feeding damage, oviposition, and the appearance of early and late instar larvae were observed for three weeks.
Adult C. distinguenda were located on both African boxthorn and L. australe during paired choice tests. Adult and larval feeding across both African boxthorn and L. australe was not significantly different. Early and late instar larvae were observed on both African boxthorn and Lycium australe.
Based on the consistent feeding damage and reproduction on the native non-target plant L. australe across no-choice, continuation, and choice tests, C. distinguenda was deemed to not be sufficiently host specific to pass a risk assessment for release.
Neoplatygaster serietuberculata – Paired choice tests (South Africa)
Lycium australe was shipped from Australia as bare rooted material to the quarantine facility at Rhodes University South Africa. Paired choice tests of N. serietuberculata adults on African boxthorn and L. australe were conducted in a quarantine facility at Rhodes University. Inside each cage, the two plants (L. ferocissimum and L. australe) were placed 10 – 15 cm apart from each other and a cardboard sheet was placed equidistant from the two plants to form a bridge that the insects could walk over from one plant to the other. At the beginning of each test, adult insects were placed at the centre of the bridge in each cage to enable the insects to orient themselves to the tested plants. The location of the insects within the cage was recorded after one hour from release. Consequently, daily recordings of location, feeding damage, and oviposition were taken for three weeks.
Adult N. serietuberculata were located on both African boxthorn and L. australe during paired choice tests during the 35-day exposure period. Adult feeding was recorded across both African boxthorn and L. australe. Oviposition occurred on African boxthorn and on L. australe, and eggs laid on L. australe were able to complete development.
Based on the high levels of feeding damage and reproduction on non-target plants in the Lycium genus, especially the native L. australe, no further testing was conducted as the insect was deemed to be not sufficiently host specific in the Australian context.
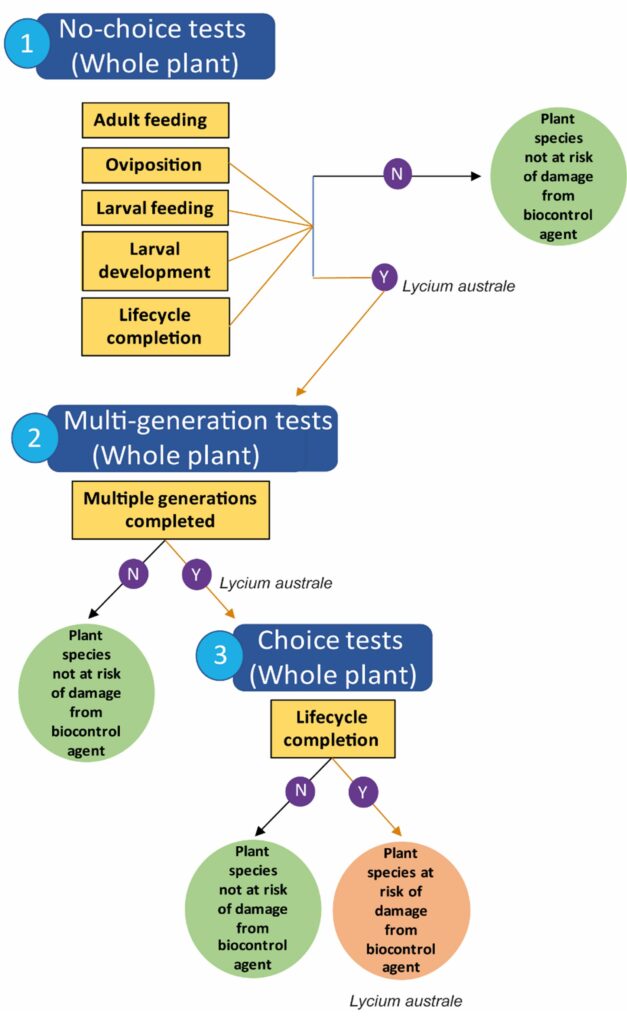
Decision tree for Cassida distinguenda biocontrol risk.
Provide a catalogue of additional candidate agents for African Boxthorn control
Native range surveys for potential biological control agents were conducted in South Africa by the staff and students of the Centre for Biological Control (CBC) at Rhodes University. Taxonomic and phylogenetic aspects of L. ferocissimum in both its native and invaded distributions were identified to ensure that potential agents were only collected from L. ferocissimum plants. More than 96 morpho-species of leaf, fruit and flower feeding arthropods were collected from over 50 sites across South Africa between 2017 and 2020. Among the insects there are 32 species of leaf feeders (Family (number of species): Chrysomelidae (11); Coccinellidae (4); Coreidae (1); Curculionidae (3); Cicadellidae (1); Coccoidea (1); Membracidae (2); Miridae (2); Pentatomidae (2); Pyrrhocoridae (1); Homoptera (1); Thripidae (2); Eriophyidae (1)), 3 species of fruit feeders (Tephritidae (2); Coreidae (1)), 1 species of seed feeder (Pyrrhocoridae (1)) and 5 species of flower feeder (Cerambycidae (1); Coccinelidae (1); Malachiidae (1); Melyridae (2)). Since then, nine stem inhabiting insects have been collected from sites in the Western cape Province. Taxonomic identifications of most collected insects were confirmed by the Plant Protection Research Institute (PPRI) Biosystematics Division – Insect Identification Service in South Africa. However, due to lack of appropriate taxonomic expertise in some insect families, many specimens still remain to be identified to the species level.
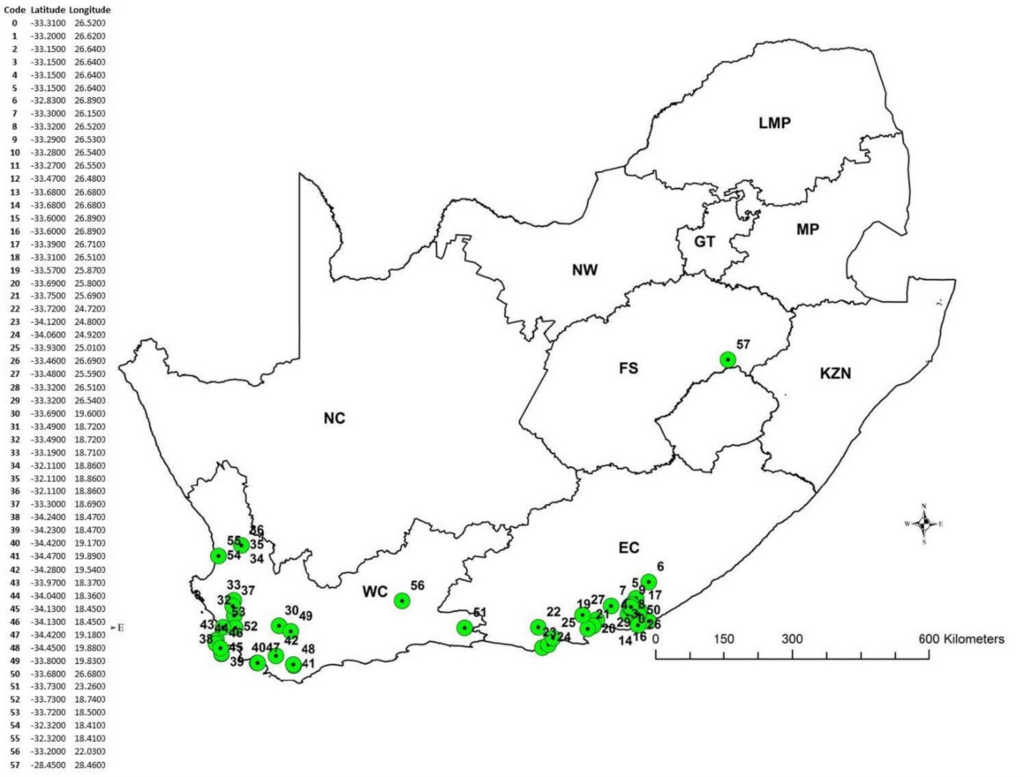
African boxthorn sites surveyed in South Africa by Centre for Biological Control. Associated site numbers and GPS co-ordinates are provided in the column left of the figure.
References
Chari LD, Mauda EV, Martin GD, Raghu S (2020) Insect herbivores associated with Lycium ferocissimum (Solanaceae) in South Africa and their potential as biological control agents in Australia. African Entomology 28(2): 359–73. https://doi.org/10.4001/003.028.0359
GBIF.org (24th July 2018) GBIF Occurrence Download https://doi.org/10.15468/dl.pbd67p.
Ireland KB, Hunter GC, Wood A, Delaisse C, Morin L (2019a) Evaluation of the rust fungus Puccinia rapipes for biological control of Lycium ferocissimum (African boxthorn) in Australia: life cycle, taxonomy and pathogenicity. Fungal Biol. 123: 811–23. https://doi.org/10.1016/j.funbio.2019.08.007
Ireland KB, Rafter M, Kumaran N, Raghu S, Morin L (2019b) Stakeholder survey reveals priorities for African boxthorn biocontrol research in Australia. Biocontrol Sci. Technol. 29:1123–28. https://doi.org/10.1080/09583157.2019.1656166
Ireland KB, Rafter MA, Raghu S, Morin L (2018) Proposed plant host test list for assessing risk of candidate biological control agents for Lycium ferocissimum. Prepared by CSIRO.
Kriticos DL, Ireland KB, Morin L, Kumaran N, Rafter MA, Ota N, Raghu S (2021) Integrating ecoclimatic niche modelling methods into classical biological control programmes. Biological Control, 160, 104667. https://doi.org/10.1016/j.biocontrol.2021.104667
Levin RA, Shak JR, Miller JS, Bernardello G, Venter AM Evolutionary relationships in tribe Lycieae (Solanaceae). In, 2007. International Society for Horticultural Science (ISHS), Leuven, Belgium, pp 225-240. https://doi:10.17660/ActaHortic.2007.745.9
McCulloch GA, Mauda EV, Chari LD, Martin GD, Gurdasani K, Morin L, Walter GH, Raghu S (2020) Genetic diversity and morphological variation in African boxthorn (Lycium ferocissimum) – Characterising the target weed for biological control. Biological Control 143:104206. https://doi.org/10.1016/j.biocontrol.2020.104206
McCulloch GA, Gurdasani K, Hereward JP, Morin L, Walter GH, Raghu S (2023). Invasion history of Lycium ferocissimum in Australia: The impact of admixture on genetic diversity and differentiation. Diversity and Distributions, 29: 879–91. https://doi.org/10.1111/ddi.13702
Noble MR, Rose M (2013) African Boxthorn National Best Practice Manual: Managing African boxthorn (Lycium ferocissimum) in Australia. Davenport, Tasmania: Tasmanian Department of Primary Industries, Parks, Water and Environment
Noble M, Adair R, Ireland K (2021). Biology of Invasive Plants 2. Lycium ferocissimum Miers. Invasive Plant Science and Management, 14(2): 41-56. https://doi:10.1017/inp.2021.13
Venter AM (2000) Taxonomy of the genus Lycium L. (Solanaceae) in Africa. University of the Orange Free State.